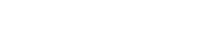
Beyond the Elements: Life
Season 47 Episode 19 | 53m 32sVideo has Audio Description, Closed Captions
Discover the fascinating chemistry that makes our world and everything in it—including us.
Without the chemistry of photosynthesis, ozone, and a molecule called Rubisco, none of us would be here. So how did we get so lucky? To find out, host David Pogue investigates the surprising molecules that allowed life on Earth to begin, and ultimately thrive. Along the way, he finds out what we’re all made of—literally.
See all videos with Audio DescriptionADProblems with Closed Captions? Closed Captioning Feedback
Problems with Closed Captions? Closed Captioning Feedback
Major funding for "Beyond the Elements: Life" is provided by the Alfred P. Sloan Foundation. Additional funding is provided by the George D. Smith Fund. Major funding for NOVA is...
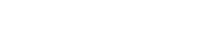
Beyond the Elements: Life
Season 47 Episode 19 | 53m 32sVideo has Audio Description, Closed Captions
Without the chemistry of photosynthesis, ozone, and a molecule called Rubisco, none of us would be here. So how did we get so lucky? To find out, host David Pogue investigates the surprising molecules that allowed life on Earth to begin, and ultimately thrive. Along the way, he finds out what we’re all made of—literally.
See all videos with Audio DescriptionADProblems with Closed Captions? Closed Captioning Feedback
How to Watch NOVA
NOVA is available to stream on pbs.org and the free PBS App, available on iPhone, Apple TV, Android TV, Android smartphones, Amazon Fire TV, Amazon Fire Tablet, Roku, Samsung Smart TV, and Vizio.
Buy Now
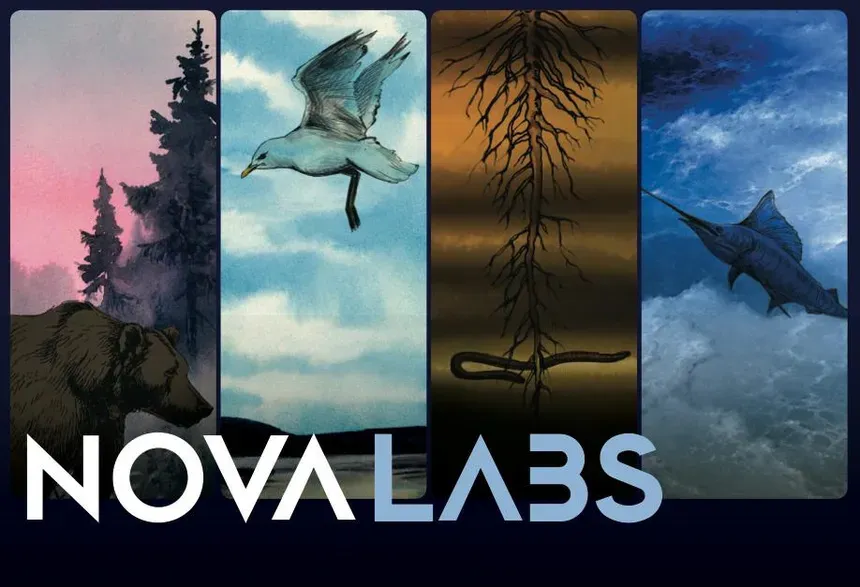
NOVA Labs
NOVA Labs is a free digital platform that engages teens and lifelong learners in games and interactives that foster authentic scientific exploration. Participants take part in real-world investigations by visualizing, analyzing, and playing with the same data that scientists use.Providing Support for PBS.org
Learn Moreabout PBS online sponsorship♪ ♪ ♪ ♪ DAVID POGUE: What's it take to make our modern world?
Ah!
(Pogue shouts) That's amazing!
I'm David Pogue.
Join me on a high-speed chase through the elements... and beyond.
(explosions) Oh, my God!
As we smash our way into the materials, molecules, and reactions... AMANDA CAVANAGH: It's a really cool enzyme because it makes life on Earth possible.
POGUE: ...that make the places we live, the bodies we live in, and the stuff we can't seem to live without.
The only thing between me and certain death... (explosions boom, glass shatters) ...is chemistry?
From killer snails... MANDË HOLFORD: Just when you think you've heard of everything, nature will surprise you.
POGUE: ...and exploding glass to the price a pepper-eating Pogue pays.
There's got to be some easier way to learn about molecules.
♪ ♪ We'll dig into the surprising way different elements combine together and blow apart.
(loud explosion) In this hour, we tackle one of the biggest mysteries of all: the origin of life.
How do a bunch of chemicals get together and start acting like a cell?
POGUE: Part of the answer might be... soap?
Cell division!
We explore how the chemistry of life transformed the planet.
LISA AINSWORTH: So everything that we eat, and the air that we breathe, all have to do with the process of photosynthesis.
POGUE: All right, so let's find the lipids aisle.
I go shopping for the macromolecules of my body.
You're telling me that that's only half the amount of fats in my body?
Absolutely.
(laughing) POGUE: And I meet an engineer who uses nature's tools to invent new molecules.
FRANCES ARNOLD: Humans have been using microbes to make beer and wine.
Instead of making sake, we make jet fuel.
POGUE: "Beyond the Elements: Life"-- right now on "NOVA".
♪ ♪ ♪ ♪ POGUE: The world we live in is made of atoms-- all listed here as elements on the periodic table.
They're the building blocks of everything around us and putting atoms together to make molecules and compounds is what we call chemistry.
And maybe the strangest event in the history of chemistry is the birth of biology.
How do a bunch of chemicals somehow get together to form life?
And if you look back at the conditions on early Earth, how did life get off the starting block?
(thunder rumbles) There was a turning point, the evolution of a transformative series of chemical reactions that, over time, remade the planet, even allowing us to come along.
But first, let's take a trip back, to Life 1.0.
(tape rewinding) So, here's the ad: Planet available.
Recently scoured by asteroids.
New construction.
Now with many water views.
♪ ♪ The atmosphere?
Eh... a fixer-upper.
(loud eruption) Devoid of oxygen.
Likely full of whatever the volcanoes spewed forth-- gases like hydrogen sulfide and methane.
(loud eruption) Somehow in that very harsh environment, primitive life got its start.
But the going stayed tough.
Food-- molecules and minerals that early organisms could use for energy-- consisted of whatever was left over from Earth's formation, or was welling up from the Earth's core at places like deep sea vents.
But in a surprisingly short amount of time-- perhaps 500 million years-- a new survival strategy evolved-- possibly the most important chemical process for life on Earth today... Photosynthesis-- the ability to turn sunlight into fuel.
Life now had access to the vast power of the sun.
In the words of one biologist, "When photosynthesis "entered the picture, life connected up to the cosmos."
♪ ♪ AINSWORTH: So, everything that we eat, what we wear, the air that we breathe, the ecosystem services that we rely on-- so things like having clean water-- all have to do with the process of photosynthesis.
It's an incredibly important, probably the most important, process on the planet.
♪ ♪ POGUE: Photosynthesis harnesses the power of sunlight to break down water molecules.
Discarding the oxygen, it uses the electrons from the hydrogen atoms to power the assembly of carbon dioxide molecules into carbohydrates, which become both building blocks and long-term energy storage.
It's not an overstatement to say that all life on this planet depends on photosynthesis.
STEVE LONG: It's exactly where all of our food comes from-- directly or indirectly.
So understanding how this process works is so important to humanity.
POGUE: Steve Long and Don Ort, two plant biologists at the University of Illinois Urbana-Champaign, along with thousands of other scientists from around the world have spent decades teasing apart the workings of photosynthesis.
Light intensity is 900 right now?
Right now, yeah.
Oh yeah.
For... that makes sense.
Sorghum is an important grain.
POGUE: Now, with that knowledge in hand, there is a new international research effort based here at the university with an audacious goal.
♪ ♪ The program's called RIPE: Realizing Increased Photosynthetic Efficiency.
WOMAN: This year was a huge hit for the canola farmers because it was too wet.
LONG: So I've been wondering whether it would be worth trying to find a substitute as our test bed.
That'd be perfect.
POGUE: These folks want to hack photosynthesis.
♪ ♪ But why would you want to do that?
Because experts think the Earth is about to get a whole lot more people.
(crowd chattering) Today, the world's population is close to eight billion.
And that's forecast to hit 9.7 billion by 2050.
♪ ♪ Raising the question: will there be enough food?
(harvester rumbling) LONG: If you look at the current rate at which we're improving crop productivity per acre of land, we're not going to get there.
♪ ♪ ORT: Part of the answer is going to be redesigning photosynthesis.
♪ ♪ (birds twittering) POGUE: To learn more about RIPE's plans, I've joined Lisa Ainsworth, a U.S.D.A.
scientist, and professor at the University of Illinois... You can see just how different the height is just in walking from one.
Wow.
POGUE: ...on an early morning tour of a field that contains 600 different varieties of soybeans.
Usually you hear about efficiency, like of a gas engine, measured in terms of a percentage, how much of the fuel is ultimately converted to energy.
What's the percentage efficiency for a plant?
Well, in terms of how much of the light energy it turns into sugar, it's pretty low-- maybe around three percent.
Three percent?!
That's terrible!
But you guys are going to help it.
That's the plan.
(chuckles) ♪ ♪ POGUE: To improve photosynthesis, two other researchers with RIPE-- Amanda Cavanagh and Paul South-- have focused on one of its key molecules.
♪ ♪ It has a very catchy name.
So the molecule is what we biologists call an enzyme.
And so it does the work.
Enzymes are like biological workers.
And the enzyme's called rubisco.
♪ ♪ SOUTH: It's R.U.B.P.-- or ribulose bisphosphate carboxylase oxygenase.
♪ ♪ CAVANAGH: And it's, for most plant biologists, one of our favorite enzymes on the planet.
Yeah, rubisco is our shortened term for it.
POGUE: Mainly because it's fun to say.
Well, it's super fun to say.
(rolling R): Rubisco.
Of course.
♪ Rubisco.
♪ But, it's also a really cool enzyme because it makes life on Earth possible.
♪ ♪ POGUE: Rubisco may not look so special but it is arguably the most important enzyme on the planet because of its critical role in photosynthesis.
Rubisco's job is to grab a molecule of carbon dioxide and feed it into a molecular machine that's building carbon chains.
That means any carbon atom that's part of any plant anywhere got there thanks to rubisco, or one of its close variants.
And because we eat plants, or animals that ate plants, that also includes just about every carbon atom in your body.
All approximately 800 million billion billion of them.
That's 26 zeroes.
Not bad, rubisco, not bad.
SOUTH: Yeah, so, if it's ever come from a plant, it had to have gone through that enzyme of rubisco.
That's wild!
Yeah.
How come there's not like a... a memorial to rubisco in Washington?
("The Star-Spangled Banner" playing) It seems, like, sort of important.
♪ ♪ Rubisco is important and that's why it's the most plentiful protein on Earth.
But just because you're important doesn't mean you're entirely... competent.
It's... in this case, not the best enzyme in the world.
It's got a hard job, so it's doing its best, but at the same time it exists in an atmosphere that's not predominantly carbon dioxide, it's mostly oxygen.
And about one in every four or five reactions it grabs oxygen instead of carbon dioxide.
♪ ♪ POGUE: That's right.
Rubisco screws up about a fifth of the time.
Instead of attaching a carbon dioxide it attaches an oxygen molecule.
And that's trouble.
You're saying nature has created a screwed-up little worker enzyme?
SOUTH: Yeah, so, 400 million years ago, when this enzyme evolved, there wasn't very much oxygen in the air.
♪ ♪ All right, so I'm the little rubisco enzyme... CAVANAGH: Yup.
and I'm like on the conveyor belt here.
Yeah.
And like, okay, carbon dioxide, carbon dioxide, carbon dioxide, carbon dioxide, oxygen and I, I don't notice I accidentally grabbed an oxygen out of the box.
(laughing) SOUTH: And it produces compounds that are inhibitory to photosynthesis, so it kind of starts to shut things down.
POGUE: I mean it's been going on for billions of years and nobody has cared.
Yeah, well...
I mean, it all... all basically works.
Photosynthesis, right now, is sort of a victim of its own success-- rubisco certainly is.
So by oxygenating the atmosphere via photosynthesis, you now have a huge amount of oxygen in the atmosphere but you need a carbon dioxide to make the reaction work.
(alarm blaring) POGUE: So what happens when rubisco screws up?
(bell ringing) The result gets shipped out through a couple other parts of the cell to where the mess is taken apart and recycled, all of which consumes a lot of energy.
If you could fix this inefficiency problem, the plant might make more... soybeans, corn, whatever it is?
CAVANAGH: That's exactly it.
Then they will have that energy to put towards something that we would consider useful like making more food for us to eat.
Is this just a crazy theory or is there some indication that this could actually work?
There's quite a bit of evidence that this is working.
So right now, we have this tested in a couple of model species.
♪ ♪ POGUE: It is tropical in here.
Amanda and Paul take me to the greenhouse to see one example.
Using two genes, one from algae and the other from a pumpkin, they've modified tobacco plants to address rubisco's sloppy work.
And why are we using tobacco plants?
Yeah, tobacco's a really useful model crop for us.
POGUE: Why tobacco?
Turns out it's one of the easiest plants to genetically manipulate, which makes it a common test subject.
SOUTH: They have definitely shown improvements in plant growth and total biomass, and we've been studying the rates of photosynthesis.
And we are pretty confident now that our model crop is successful in this pathway, and now we're really interested in moving these into something we like to eat.
♪ ♪ POGUE: Reducing the energy penalty crops pay for rubisco's mistakes could be huge.
In soybeans, a 25% reduction could result in plants that produce more than 60 million more bushels a year.
CAVANAGH: This to a lot of people is an idea that might be out there, but if we can get it, if we can get this moonshot approach to work, then we're going to have more food.
And so that's really what drives what I do.
♪ ♪ POGUE: The RIPE program is international.
And, likely, so will be the reach of any of its discoveries.
♪ ♪ But work like theirs is not without controversy.
Some of RIPE's solutions depend on cross-breeding plants chosen for their genes.
But other solutions, like the rubisco work, depend on genetic engineering-- also called genetic modification, or GM-- adding new genes from other types of plants, or even organisms, entirely.
The laws governing genetically modified crops vary from country to country, especially when it comes to labeling their use in food.
(indistinct chatter) And there have been objections to some companies that patent their new crops and control who can plant them.
But the general scientific consensus is that they are no more dangerous than conventional crops, though they need to be carefully studied for potential health and environmental effects.
The U.S., unlike Europe, has largely adopted GM plants.
An overwhelming percentage of corn, soybeans, and cotton grown in the United States is genetically modified.
♪ ♪ LONG: I understand there are concerns.
As a scientist, I feel those concerns have very little validity, although clearly people have become very concerned-- particularly in Europe.
Of course, in this part of the world, genetically modified crops have been grown for over 20 years.
This technology has spread throughout the Americas.
♪ ♪ POGUE: In fact, as the global population grows, it's in poorer countries that RIPE's work may end up having the greatest impact.
Especially if genetically modified foods gain acceptance.
LONG: The place where I see the technology needed most is actually in sub-Saharan Africa.
And this opposition to GM is having quite an influence in Africa.
It's keeping the science, which is needed, out and I fear that this could risk people starving when we could actually be giving them seed which would allow them to feed themselves into the future.
♪ ♪ POGUE: Even if scientists succeed in improving photosynthesis, it won't have anywhere near the dramatic impact of the original version introduced about three billion years ago.
Back then, scientists believe, photosynthetic cyanobacteria began cranking out oxygen as a waste product.
Eventually, bacteria produced enough oxygen that it started to accumulate in the atmosphere.
Which, in turn, gave rise to one of life's underappreciated molecular allies-- the ozone layer.
It's in the lowest level of the stratosphere, between roughly eight and 22 miles up.
♪ ♪ Atmospheric research planes venture up here... (radio chatter) ...but not much else.
♪ ♪ The ozone comes from a process even higher up in the stratosphere.
There, solar radiation busts up O2 molecules into individual oxygen atoms.
They drift down to the ozone layer where they convert O2 into O3-- ozone.
Despite the name, there's not that much ozone in the ozone layer-- less than ten parts per million-- yet it's had a profound effect on the evolution of life on Earth.
♪ ♪ To find out more... POGUE: So ozone is O3, right?
KERRY HANSON: Ozone is O3.
POGUE: ...I travel to the University of California, Riverside, to meet Kerry Hanson... We came alive because... POGUE: ...a research chemist who studies how molecules like ozone and those in sunscreens interact with light.
So any molecule can absorb light.
POGUE: It turns out the ozone layer and sunscreens have a lot in common.
This O3 gas is out there in the atmosphere in such quantity that there's an envelope around the whole planet?
Yeah.
It's a layer.
Think of like a sunscreen.
You know, how we use sunscreen on our skin?
Yeah.
So it's the exact same thing.
The ozone layer is earth's sunscreen.
♪ ♪ POGUE: Both the ozone layer and sunscreens protect us from the harmful effects of ultraviolet radiation-- or U.V.-- a kind of sunlight that, unlike the colors of the rainbow, we can't see.
♪ ♪ On the electromagnetic spectrum, visible light sits here, but U.V.
sits up here at a higher energy.
Scientists divide it roughly into three kinds-- A, B, and C. And while A and B, aren't good for you-- and they're the reason to wear sunscreen-- it's C that's the big problem for living things because it's particularly destructive to DNA.
♪ ♪ (players shouting) Kerry tells me how all this relates to ozone.
Just another Sunday watching volleyball.
(voiceover): And it's kind of like volleyball.
♪ ♪ Oh!
He's good.
POGUE: Well... if the balls were different kinds of U.V.
In the early days of life on Earth, before photosynthetic bacteria oxygenated our atmosphere... Get it, get it, get it... yeah, yeah, yeah!
♪ ♪ There was no ozone layer and no global defense against ultra violet radiation.
Aw... ooh...
The most dangerous kind, U.V.C., bathed the planet, which may have effectively limited where life could grow.
But oxygen accumulating in the atmosphere and the rise of the ozone layer changed all that.
The layer blocks all the U.V.C.
and most of the U.V.B.
from reaching the Earth's surface.
HANSON: Oh... good block!
POGUE: Here's how it works: when U.V.
radiation hits a molecule of ozone, it splits it into an oxygen atom and a molecule of O2.
The U.V.
light has been absorbed and neutralized.
The lone atom quickly rejoins another molecule of O2 to reform ozone.
The net result is a conversion of that harmful radiation into heat.
Despite the ozone layer, we can still get hit by unhealthy amounts of U.V.
And that's why it's a good idea to use sunscreen.
HANSON: If you read the label, and if it says broad spectrum, that means it's blocking U.V.B.
and U.V.A.
Wow.
Not U.V.C., like ozone, but U.V.A.
and B.
♪ ♪ Just like we use sunscreen to block harmful U.V.A.
and B radiation from our skin... the ozone layer protects planet Earth from harmful U.V.C.
radiation... that would destroy the building blocks of life-- DNA.
Without the blocking of U.V.C.
by the ozone layer, life would not have been able to come out of those oceans, come up on to land, and you and I wouldn't be talking here today.
POGUE (echoing): Thanks, ozone!
♪ ♪ Without that global protection, the grand story of evolution that began from single-cell ocean-dwelling life and led to the wondrous complexity of multicellular animals occupying land, sea, and sky would probably never have been told.
(film reel clicking, score stops) (grunts) And yada yada yada... Yeah, yeah, I know, the evolution of life is important, but let's talk about something really important-- me!
Or at least me and my molecules.
I know what elements I'm made of: CHNOPS-- carbon, hydrogen, nitrogen, oxygen, phosphorus, sulfur-- CHNOPS!
There are other elements in the human body, but these are the main six.
And, of course, a good chunk of me by mass is good old H2O.
But if you take that water away... most of what's left is macromolecules-- mostly big, long polymers-- chains of smaller molecules.
Yeah, so I once went "CHNOPing."
That's C-H-N-O... (voiceover): To learn more about them, biologist Monica Hall-Porter-- formerly at Lasell University, now at the University of Texas-- offers to show me around a local... supermarket?
♪ ♪ It's kind of weird, I ask you about the molecules of my body, and you bring us to a grocery store.
Yeah, so today's shopping trip is about the macromolecules that actually make up the human body.
♪ ♪ Specifically protein, lipids or fats, carbohydrates, and nucleic acid.
And if you take a look around the grocery store, there are many examples of those macromolecules here.
All right, show me the ropes.
Let's go shopping.
♪ ♪ (laughing) POGUE: Our first objective?
Protein molecules.
Monica tells me, by weight, that's about 20% of my body.
Does that mean pure masculine muscle?
Is that what you're saying?
Well, not necessarily muscle.
♪ ♪ Proteins are the molecules that actually do work in cells.
So not just composing muscle but also the proteins that serve as the structural proteins in our hair, fingernails.
POGUE: The most abundant protein in your body is collagen, making up fibrous tissues like skin, tendons, and ligaments.
There's also collagen in teeth and bone.
But even though there are tens of thousands of different proteins in the human body-- maybe millions, no one is sure-- amazingly, they're all made from stringing together about 20 different kinds of small molecules called amino acids, which we get by breaking down the proteins we eat in a variety of foods.
HALL-PORTER: And so when we consume protein, like in turkey for example... (chortling): Whoa!
Our body breaks the amino acids down and then the amino acids are incorporated into proteins that our bodies synthesizes or makes.
Yep, there you go!
Handsome little gobbler.
POGUE: Next on the macromolecule shopping list: lipids.
All right let's find the lipids aisle.
Well, there's no lipids aisle, (laughs) but we can get oils and fats.
So let's head down this way and let's get some oil.
This is massive, how much fats are we getting?
A lot.
POGUE: Oh, man.
POGUE: This seems like we've got 15 pounds of fats here.
Yes.
And you're telling me that's only half the amount in my body?
Absolutely, you're about 30 pounds of fat.
Now, I have to say I find that a little insulting.
Well, you shouldn't.
♪ ♪ POGUE: Compared to proteins, lipids or fats do get a bad rap.
But in addition to their role in cell membranes, and long-term energy storage-- you know, body fat-- they also provide protection for internal organs.
Oh, and don't forget the lipids in earwax!
HALL-PORTER: And so literally there's fat in every part of you.
So even a slim, lean, handsome, physically fit person could have thirty pounds of fat in him?
Absolutely.
♪ ♪ POGUE: Next up, the third most common macromolecule type... Oh, this is my kind of food group.
Carbohydrates.
Sugar!
Sugar!
Does this count as carbs?
Absolutely.
POGUE: While I would have thought I was sweeter, turns out on average there's only about two pounds of carbs in me.
Glucose is the most abundant carb in the human body.
It circulates to provide energy for cells.
Now we're talking carbs-- carb city, carb heaven, carb central... We are in the bread aisle, my friend.
I like it!
All right, we can toast this up and put some butter on it... (laughs) POGUE: Many glucose molecules joined together can make a plant starch, the kind you find in cereals and root vegetables.
It's the most common carb in the human diet.
So did I under... (indistinct voice over loudspeaker) Hey, we're workin' here!
So we've got lipids.
Yeah.
Proteins.
Yeah.
And carbohydrates.
Yeah, the three macromolecules of the human body.
Right, but we're missing one.
There's another one?
Yeah, we don't have anything that's representative of nucleic acids.
POGUE: Nucleic acids are better known as DNA and RNA.
DNA is the famous double helix.
It's usually two long chains of molecules that wrap around each other.
It contains genetic instructions for making proteins.
RNA is often a long molecular chain as well.
If DNA is the cookbook, RNA is the chef, reading DNA's instructions for proteins, gathering the ingredient amino acids, and assembling them in the right order in a macromolecular protein printing machine called a ribosome.
Life on Earth exists in a spectacular variety of forms, but in the end, it all depends on the arrangement of a handful of different small molecules-- the nucleotides-- in the nucleic acids DNA and RNA.
And we are now arriving at the DNA aisle.
All righty.
And why strawberries?
Well, strawberries actually have eight copies of each chromosome per cell.
So relative to other fruits, strawberries are actually very rich in DNA.
Wow, all right.
Here's our DNA-ey berries.
♪ ♪ Actually seeing DNA-- you know, the code of life-- has always seemed beyond the reach of ordinary folks.
You can't just find some, can you?
When you said we were going to extract DNA from strawberries, I figured we would go to some humming, high-tech lab with millions of dollars of equipment... (maniacal laughter) No, actually DNA extraction from strawberries is something that can be achieved at home.
POGUE: As it turns out, using some easily available household items like plastic bags, detergent, rubbing alcohol, cheesecloth and strawberries, (liquid dripping) along with a little bit of waiting time, you too can catch a glimpse of the code of life-- DNA.
♪ ♪ There it is.
You'll see an accumulation of white, stringy substance.
That's actually a very crude prep of DNA.
♪ ♪ Basically, what's going to happen is, it's going to clump on the end of your glass rod.
Strawberry DNA slime, right there.
(laughs) ♪ ♪ POGUE: Pretty amazing.
And so are the other three macromolecules that make up my body.
But all their wondrous complexity raises a deeply mysterious question: how did chemistry give rise to biology?
How did life get its start?
♪ ♪ A famous experiment in 1952 suggested the answer might not be that hard to find.
At the University of Chicago, graduate student Stanley Miller, with help from his doctoral advisor Harold Urey... (gas hissing) Mixed what were then thought to be the dominant ingredients of Earth's early atmosphere-- methane, ammonia, and hydrogen-- inside some sealed glassware.
Boiling water added water vapor to the mix.
Then Miller created sparks between electrodes simulating lightning, and let the mixture cool and condense.
After running the experiment for a week, Miller found five amino acids... some of them critical building blocks of proteins.
JACK SZOSTAK: You know, it was a dramatic breakthrough at the time for people to realize that amino acids could be made in such a simple way.
♪ ♪ POGUE: At Massachusetts General Hospital, Jack Szostak runs one of the several research labs around the world that are trying to figure out how chemistry gave rise to biology.
So this is like increasing the amount of sodium hydroxide, and so increasing... Oh, okay.
POGUE: Today, it's clear even the Miller Urey experiment, while groundbreaking, just scratched the surface of the problem.
SZOSTAK: In retrospect, it kind of fooled people into thinking that the answers might be easier than they turned out to be, right?
Once you've got the right chemicals, then what?
POGUE: Right, right.
How do a bunch of chemicals get together and start acting like a cell?
♪ ♪ POGUE: A key requirement seems to be a container.
All life on Earth, from the simplest to the most complex, is made of cells, with "outer membranes."
So on the road to life, how did that happen?
♪ ♪ Scientists like Anna Wang, a former post-doc in Jack Szostak's lab, now a professor at UNSW Sydney, have been working with a simple molecule that is one of the prime suspects.
It's also present here.
Wow.
♪ ♪ POGUE: Shaped into bars in a wide variety of colors and scents.
Smells good in here.
Smells amazing.
POGUE: At Molly's Apothecary outside of Boston.
Oh, that's wonderful.
POGUE: That's right... soap!
Soap's interesting because a soap molecule is a combination of two different types of molecules, called polar and non-polar.
♪ ♪ For example, water molecules are polar.
Each one has a concentration of electrons in one part, making it negative, which leaves another part more positive.
That's polarity.
And it makes water molecules want to stick together, each negative part attracted to another molecule's positive part.
An oil molecule, made up of carbon and hydrogen, is an example of a non-polar molecule.
It has an even distribution of electrons-- no polarity.
And less stickiness between molecules.
In fact, polarity is why oil and water don't mix.
The polar water molecules stick together, keeping the oil molecules at bay.
The less dense oil floats on top.
That's also why trying to clean oily grease off your hands with water alone, doesn't work very well.
It actually won't come off, it's super oily.
The two just don't interact.
♪ ♪ And that's where soap molecules come in.
They're hybrids; at one end are some negatively charged, electron-rich oxygens, ready to interact with polar molecules like water, but the rest is a long, non-polar hydrocarbon tail, with no positive or negative charge.
It's more comfortable mixing with other non-polar molecules, like grease.
Put some soap on your greasy hands... Soapy!
And the soap's non-polar tails stick into the grease while its polar heads act like handles, ready to interact with the water taking the grease along for the ride.
Here's another interesting soap fact.
Drop some soap into water, and the molecules form little balls called micelles, with their water-loving polar heads sticking out, and their water-hating non-polar tails sticking in.
That naturally occurring little container has piqued the interest of scientists like Anna.
♪ ♪ Back at the lab, she adds some soap molecules to water containing short fragments of RNA.
They've been tagged with a molecule that makes them glow.
Why RNA?
The current scientific consensus is that a primitive form of RNA may have been the first molecule with the ability to replicate itself, jump-starting evolution.
Next stop... Now we're going to go look at it under the microscope.
POGUE: The microscope room... where Anna loads up a sample she prepared yesterday.
WANG: So this is what our soap molecules have self-assembled into overnight.
POGUE: What are they, bubbles?
WANG: Yeah, they're almost like bubbles and so what we're looking here is not the soap molecules themselves but what they've been able to trap inside these cell-sized structures.
POGUE: Overnight, the soap micelles have self-assembled into larger spheres, trapping the fluorescing RNA inside.
And if we could zoom into one of them... we'd see that it actually consists of two layers of soap molecules, arranged with the water-loving heads toward the inside and outside, and the water-hating tails brought together.
When you have molecules that have a polar head group and a non-polar tail but you don't give them any oil to interact with, the oily tails actually want to interact with each other.
And so you end up forming these bilayer structures.
Wait, so these are soap molecules and these are also soap molecules?
Yeah.
And they like to... assemble into this position?
Yeah, that's right, so they like to form these really thin envelopes, and you can imagine this structure extending onwards and onwards and curving around and forming a sphere.
And that's what we're seeing here.
We're seeing this bilayer structure encapsulating some green-dyed RNA molecules.
POGUE: This lipid, bilayer structure isn't alive, but it's familiar to biologists.
It's similar to the bilayer structure of the membranes that surrounds something that is alive-- cells.
Of course those are much more complicated and more stable containers, better at keeping things in or out, though that feature comes at a price.
If you take the membranes that we have now but get rid of all the highly evolved protein machinery, what you're left with is just an inert sack.
It can't grow, it can't divide.
It can't even get nutrients in and out.
POGUE: That's why in the days of proto-life, less-stable membranes built out of simpler molecules like soap may have been an advantage.
Anna shows me an example.
So what I'm about to do is I have some soapy water in here and I'm just going to add it.
♪ ♪ WANG: What happens is those soap molecules start incorporating onto the existing membrane... Look at this, look at this!
Yeah!
(chuckles) It just split!
So they look pretty spherical now, but they're starting to wiggle a bit.
And all of a sudden... it looks like they might melt.
Cell division!
POGUE: Wow.
Our cells grow and divide because we have something giving instructions.
Yes.
But you're saying that billions of years ago, none of that existed.
There's none of that in here, so what we're kind of simulating is a condition where maybe these protocells have floated somewhere down the stream, and they've come across a pool of excess soap molecules, and these soap molecules can join the membrane and grow it.
So I think what it means is that we can still get simple cells to divide by purely physical mechanisms, and that's what we're trying to understand in the field, like how do you get to do things that kind of seem like life and mimic life but without any biology?
♪ ♪ POGUE: In the early days of Earth, soap or similar molecules may have self-assembled into cell-like containers... POGUE: Did they have the bilayer thing already?
They have a bilayer membrane.
Yeah.
POGUE: But Jack Szostak realizes that's just a start.
There are many more steps on the road from chemistry to biology.
Once you got the right kinds of molecules, which are pretty simple, they can assemble into membranes.
But they can't actually start to do anything interesting in terms of, like, getting more complicated and being more, like, more and more advanced life until you have genetics.
Yeah.
SZOSTAK: You need a hereditary material, something like RNA or DNA.
And once you've done that, you have cycles of replication, because that's got to go on inside these protocells.
And it's got to happen just by chemistry and physics because there were no enzymes.
There was no evolved machinery, right?
So, in a sense, the answer has to be simple.
Yeah.
And we just have to figure out how it works.
(chuckling) ♪ ♪ POGUE: Scientists like Jack and Anna are searching for the mysterious road that led not only to life but to the mechanism that's allowed it to overcome adversity-- evolution.
♪ ♪ Today, some scientists wonder what if we could harness evolution's creative power to solve some of our own challenges?
Wow.
FRANCES ARNOLD: Nature is constantly changing.
Hi, boys.
Because there is this tremendous effort to survive.
If you can harness that power, that innovation that nature is doing and direct it in a beneficial way, then we can use that power of innovation to solve some of our really tough problems.
POGUE: Harnessing the innovative power of evolution is at the heart of the work of chemical engineer Frances Arnold of Caltech in Pasadena, California.
That could be a huge deal in the world.
I hope so.
POGUE: And she's used it to engineer new molecules to solve a wide range of problems.
From the search for new antibiotics, or methods to convert waste into biofuels, to teaching cells to bond elements in ways never before seen in nature.
ARNOLD: So do come if you're interested in the process of protein engineering, because that's the future, so all of you who are going to do... POGUE: She's achieved her successes by discovering new catalysts, the materials that speed up chemical reactions without getting consumed by them.
In C edge functionalization... POGUE: In living things, catalysts are called enzymes-- for example, the protein rubisco.
Enzymes help facilitate the reactions that make life possible.
ARNOLD: The reason that you and I can sit here and talk is that we have thousands of catalysts in us, proteins, that can convert the food we eat into the thoughts that you think... and the motor mouth, right?
(chuckles) These are catalysts that do all this chemistry.
These are chemical transformations that make life possible.
♪ ♪ POGUE: In fact they work so well, engineers and scientists have wanted to find a way to co-opt the idea, to create new enzymes that would do our bidding, assisting reactions that aren't found in nature at all.
The question is how?
ARNOLD: Many scientists and engineers feel that in order to design a new product, you sit down and you calculate, you know, the right angles and the right weights and loads.
I come from a different point of view, that these very complicated things are the products of evolution.
So I say, "Let's just go straight to the answer, using this gift given to us."
♪ ♪ POGUE: Frances uses an approach called directed evolution.
ARNOLD: One way to think about directing evolution, is it's like breeding.
It's like breeding cats or dogs.
♪ ♪ POGUE: With a specific end goal in mind, She starts with DNA that encodes for some protein catalysts that have some promising traits, depending on what she's looking for.
The DNA gets copied in a way that produces random mutations.
She puts that into microorganisms that multiply and produce a variety of slightly different proteins.
ARNOLD: So you have a gene, the organism reads the gene, makes the proteins, and they're all slightly different, just like your children... (laughs) but now I can decide who goes on to parent the next generation because I measure what those proteins do.
POGUE: Frances tests the results to see if any represent a step in the right direction.
If so, that becomes the new starting point, and she repeats the process.
ARNOLD: To see how quickly you can train enzymes, that's what we're doing, we're training them, we're breeding them... to do something that perhaps nature never did before.
When you discover that they've learned how to do that and they do it better than any human can do, it is so exciting.
♪ ♪ POGUE: To see how directed evolution can work outside the lab...
Farming has always been about increasing productivity.
POGUE: ...Frances suggested that I contact one of her former students, Pedro Coelho.
Along with a partner, she and Pedro founded a company Provivi, based in Santa Monica.
Pedro is the CEO.
Provivi makes a chemical to fight this agricultural pest, the fall army worm.
COELHO: It's a pest that is native to the Americas, but in the last three years, it's invaded all of Africa and now, all of Asia, going from India to China, and it's a very difficult pest to control because once it infests the corn, it hides inside the corn plant where the insecticides can't touch it.
♪ ♪ POGUE: But Provivi's chemical isn't a pesticide, it doesn't kill anything.
Instead it disrupts the way fall army worms mate.
Here's how it works: Fall army worms eventually become adult moths, and that's when they mate.
To attract males, female moths release a pheromone, a molecule that acts as a chemical signal.
So the female moth will release a little bit of pheromone, and then the male will pick up that signal with his antenna and will fly towards her to mate and reproduce.
POGUE: She uses only a small amount, but it's incredibly potent.
It can attract males from up to a mile away.
ARNOLD: These are complicated molecules.
These are the Chanel No.
5 of insects.
(laughter) POGUE: But such a powerful "sex perfume" can become a means of control.
So imagine, now, you come with a bottle of Chanel No.
5 and you spray it everywhere.
(spritzing sound effect) ARNOLD: Then he can't find her, and they don't mate and have caterpillars.
♪ ♪ POGUE: Provivi has figured out how to replicate the fall army pheromone, and put it into a slow release spray for crops.
Which you have to imagine is very confusing for the male moths.
They have so much trouble finding females that in the end there are fewer eggs, and worms.
So you're not killing these things, and you're not driving them away, you're just confusing them?
Yeah, so it's not a repellant, and it's not a kill agent, it's simply a mating disruptor.
♪ ♪ POGUE: Pedro tells me using pheromones to combat pests isn't new.
But until now, it's been expensive, and therefore limited to high-value smaller crops like apples or grapes.
So the real breakthrough at Provivi isn't using pheromones but making them inexpensively.
They've studied the enzyme catalysts the insect uses to make the pheromone, and moved the genes for those enzyme catalysts into yeast.
Then, through directed evolution, they optimize those little yeast cell factories for larger scale production in vessels similar to those used for brewing beer.
(machine beeps) COELHO: And the key is that by just changing the microbe, we can make many different pheromones but using the same infrastructure, which gives us the economies of scale and should make this cost effective.
POGUE: Making it possible to use on staple crops grown around the world, like corn, and rice.
COELHO: Our mission very much is to take this proven tool of pheromones to the largest markets of agriculture, which are the staples of humankind.
♪ ♪ POGUE: Companies like Provivi aren't the only sign that directed evolution and cell factories are having a big impact on manufacturing.
Well, I teach this course called Reaction Engineering which is how do you take chemical reactions and scale them up?
POGUE: In 2018, Frances Arnold won the Nobel Prize in Chemistry, for her pioneering work in directed evolution.
Is this idea of chemistry and biology to manufacture stuff, is that catching on these days?
It is, it most definitely is.
I think the future is so exciting, because now what happens is with these tools of being able to manipulate DNA, and the code of life, really, we can now merge all these beautiful mechanisms of the biological world with the inventions of human chemistry.
And that way, it merges in new innovations.
♪ ♪ POGUE: That both chemists and biologists have a lot to learn from each other should come as no surprise.
But what is surprising is that biology would arise out of chemistry at all.
Look at this, look at this!
Cell division!
(dramatic voice): The blueprints of life!
The origin of life remains one of the great unsolved mysteries of science.
Was the mix of chemicals on early Earth destined to give rise to life?
And once it started, was the road that lead to the chemical complexity of photosynthesis and the harnessing of the power of the sun...
Probably the most important process on the planet.
POGUE: ...the only road to be taken?
Are we alone in the universe?
Or just the local branch of cosmic bio-chem?
The answers to questions like these will be found only through science... as we go beyond the elements.
♪ ♪ ♪ ♪ ANNOUNCER: To order this program on DVD, visit ShopPBS.
Or call 1-800-PLAY-PBS.
Episodes of "NOVA" are available with Passport.
"NOVA" is also available on Amazon Prime Video.
♪ ♪ ♪ ♪
Beyond the Elements: Life Preview
Video has Closed Captions
Preview: S47 Ep19 | 30s | Discover the fascinating chemistry that makes our world and everything in it—including us. (30s)
Growing Simple Cells with the Help of... Soap?
Video has Closed Captions
Clip: S47 Ep19 | 7m 11s | To jumpstart evolution, a scientist shows, just add soap. (7m 11s)
Meet the Enzyme Driving Photosynthesis
Video has Closed Captions
Clip: S47 Ep19 | 6m 8s | Hear from two scientists trying to hack photosynthesis. (6m 8s)
Providing Support for PBS.org
Learn Moreabout PBS online sponsorshipSupport for PBS provided by:
Major funding for "Beyond the Elements: Life" is provided by the Alfred P. Sloan Foundation. Additional funding is provided by the George D. Smith Fund. Major funding for NOVA is...